To address all objectives, a suite of modeling tools is needed, which includes a DNS cloud model, WRF regional climate model with an updated aerosol module, WRF cloud-resolving model, cloud seeding bulk and bin microphysics schemes, and piggybacking method. The brief description of these modeling tools and the numerical experiments are provided in the following:
Available modeling tools
The DNS cloud model that is used in this project consists of two parts: turbulence dynamics and cloud droplet microphysics. This model was initially developed in McGill University in the late 1990’s and early 2000’s (Franklin et al. 2005; Vaillancourt et al. 2001) and has been continuously developed and improved since then (Franklin et al., 2007; Franklin 2014; Chen et al. 2016). The turbulence model explicitly solves the incompressible Navier-Stokes equations (vorticity form) in a domain with triple periodic boundary conditions. The dynamics is explicitly resolved on scales greater than the Kolmogorov scale (Kolmogorov 1962) based on the eddy dissipation rate (EDR). The cloud microphysics model tracks trajectory of individual cloud droplet in a Lagrangrian framework driven by the turbulent velocity fields and only considered the collision-coalescence process without condensation given an initial cloud droplet PSD until recently. Disturbance flows induced by cloud droplets were superimposed onto the turbulent flows so that the impact of such flows on collision efficiency among droplets can be studied (Rosa et al. 2011; Wang et al. 2005; Alyala et al. 2007, 2014; Chen et al. 2016). Most recently, Lulin Xue and Sisi Chen worked together to implement the cloud droplet condensation process into the model and simulated the complete growth history of cloud droplets in DNS for the first time (Chen et al. 2018). Figure 1 shows an example of the initial cloud droplet locations on the left, and initial PSD (black), PSDs at 5 minutes for simulations just consider collision-coalescence (yellow), condensation (red), and both processes (blue) simulated by the DNS cloud model on the right. The results clearly show that in turbulent environment both condensation and collision-coalescence processes quickly broaden the spectrum to initiate warm rain. The implementation of aerosol activation and wet-growth based on the hygroscopicity of individual aerosol particle is completed. The hygroscopic effect on cloud and rain formation is being studied using the DNS cloud model for the first time in the UAE-NATURE.
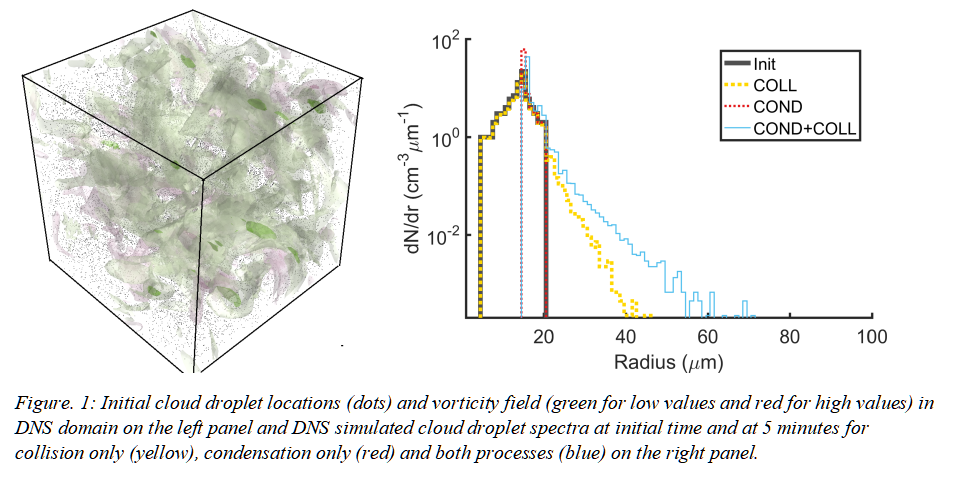
The WRF model is the most popular mesoscale atmospheric model by both operational and research communities to provide forecast services and conduct scientific research (Skamarock 2008). We will use WRF for both the high-resolution regional climate simulation and the cloud seeding sensitivity simulations in this proposed study. On the regional climate simulation side, the newest version has the following features that are suitable for this task: 1) time varying lower boundary conditions (such as sea surface temperature, leave area index etc.), 2) time varying concentrations of greenhouse gases, 3) hybrid vertical coordinate for improved simulation in complex terrain, and 4) bucket options for more accurate accumulated precipitation and net energy flux. On the cloud seeding simulation side, WRF improved the moist dynamics for Large Eddy Simulation (LES) that will be used for idealized sensitivity simulations. Advanced microphysics schemes that are suitable for aerosol-cloud interaction and cloud seeding impact are available to WRF.
University of Pécs and NCAR Bin scheme has been developed jointly between UP and NCAR since late 1990s (Geresdi et al 1998, 2005, 2014; Rasmussen et al 2002; Xue et al. 2010, 2012). In bulk microphysics schemes, only the total mass and/or number mixing ratios of different hydrometeor species are predicted. The size distribution of each species is often parameterized by prescribed functions. Unlike the bulk approach, “bin” microphysics divides hydrometeors into many discrete diameter or mass bins and predicts the mass and/or number mixing ratios for each bin. Since no size distributions of hydrometeor species are prescribed, the cloud can evolve and respond to hygroscopic or AgI seeding in a more realistic way in a bin scheme. Another advantage of a bin scheme is that the simulated results can be directly compared to the in-situ observed PSD. The UPNB scheme calculates the number and mass mixing ratios of four different types of hydrometeors (water drops, pristine ice crystals, snowflakes and graupel particles) in 36 mass (size) bins. This method allows the model to simulate the explicit cloud PSD, such as those in Figure 1. We will develop aerosol and hygroscopic seeding modules for this scheme. Glaciogenic seeding mechanism similar to the one in Xue et al. 2013a has been coupled to UPNB (Geresdi et al. 2017). A cloud electrification parameterization for UPNB is under development based on the work of Xu et al. (2014, 2016).
Microphysical piggybacking method was developed to assess the quantitative impact of microphysics on simulated storm macrophysics such as the rainfall amount and distribution (Grabowski 2014, 2015). The problem is especially difficult in the case of a convective cloud because of a tight coupling between cloud microphysics and highly nonlinear cloud dynamics. In a nutshell, two simulations with even slight modification of the cloud microphysics (or any other element of the simulation, like the initial conditions) diverge relatively quickly and separating the microphysical impact from the impact of natural variability is impossible. The natural variability also implies that effects of cloud seeding are difficult to assess in observations and in cloud modeling. We plan to use the piggybacking methodology in the proposed project. The key idea behind microphysical piggybacking is to use two sets of thermodynamic variables (the potential temperature, vapor mixing ratio, and all variables describing cloud and precipitation particles) in a single simulation. One set is coupled to the dynamics and drives the simulation, and the second set is applied as in the kinematic model, that is, piggybacking the simulated flow but not affecting it (see Figure 2). In the case of cloud seeding simulation with piggybacking, one set of variables will include effects of seeding and the other set will not (i.e. 1st step in Figure 2). Since only one set of variables will drive the simulations, the two sets (i.e., with and without seeding) will simulate cloud processes in exactly the same cloud-scale flow. It follows that comparing precipitation predicted by the two sets of thermodynamic variables will demonstrate purely microphysical effect. To investigate a possible dynamical feedback, one complements the simulation with another one, where the set driving the simulation is switched with the one piggybacking it, and vice versa (2nd step in Figure 2). The second simulation typically involves a different flow realization, but one can still compare the change between the driving and piggybacking sets in the two simulations. If the change is very different, then one can argue that it makes a difference in dynamics (say, seed vs. noseed). As shown in Grabowski (2014, 2015) and Grabowski and Morrison (2016, 2017), a small ensemble allows assessing the microphysical impact and separating the microphysical impact from the dynamical effect. Microphysical piggybacking will be an essential component of the modeling part of the proposed project. It allows accurate assessment of the microphysical effects and separation of microphysical and dynamical impacts, both playing the key role in the evaluation of cloud seeding impacts.

DNS cloud and rain formation simulations
The DNS cloud model will simulate the cloud evolution in a realistic condition to provide the quantitative data for parameterization development in WRF.
Due to the limitations of the disturbance flow assumption and the stability requirement, the droplet radius is limited to 100 microns in the DNS simulation. Therefore, only the warm rain initiation and associated hygroscopic seeding impact can be effectively studied using this modeling tool. The DNS cloud model will be setup to just simulate the core or the least-diluted region of the chamber-formed clouds with a volume of several liters. The time step is very small at the order of 10-4 s to integrate all microphysical processes, track droplet positions accurately and maintain the stable calculation of disturbance flows around droplets. The solute effect and curvature effect on diffusion growth of wet aerosol particles are explicitly calculated. The laboratory measurements will be compared with the DNS results. Some assumptions of the aerosol solubility and mixing state may be adjusted in the model to better reproduce the chamber results. Once the DNS qualitatively agree with the chamber results, the DNS cloud model will be configured to simulate cloud and rain formation in convective conditions. By varying the updraft speed, ambient thermo-dynamic condition, turbulent strength and seeding scenarios, the warm rain initiation relationship in the multiple parameters space can be derived, which will be parameterized into microphysics schemes for regional climate and cloud seeding simulations.
The DNS cloud simulations address objective 1 in this proposed research.
High-resolution regional climate simulations
The proposed 10-year high-resolution regional climate simulation will use single domain with 9 km grid spacing to capture both the extratropical influences from the midlatitude eddies and the subtropical moisture sources over the Red and Arabian Seas. The model will be run without convective parameterization so that the convective and microphysical process will be explicitly simulated. A smaller inner domain will cover the Gulf region at a finer grid spacing for summertime precipitation simulations. The higher resolution is motivated to capture the dynamics of mesoscale system over the Arabian Gulf, which is often associated with extreme rainfall over the Gulf.
The NYUAD has previously used a similar configuration to successfully study the evolution of rainfall over the Indian Ocean and Monsoon regions (Chen et al. 2017a, b). Rainfall in the UAE is strongly influenced by extratropical weather systems and topography. In addition, due to the combination of desert climate and highly populated urban area, the region exhibits a complex array of natural and anthropogenic aerosols. These factors motivate the use of a high-resolution model that can both capture the fine dynamical structures and included a detailed representation of the cloud microphysical processes.
The implement of a new aerosol scheme including dust and sea salt will represent the aerosol background in UAE. ERA5 was determined to be the best forcing to drive the long-term simulations. Different model physics will be tested using the best reanalysis data. The 10-year results will quantify the natural variability of rainfall in both space and time over the UAE. The large and synoptic scale patterns associated with specific rainfall types or events can be identified. The continuous meteorological data simulated by the model can also be used for many fields other than cloud seeding, such as agriculture, ecology, hydrology, traffic weather, renewable energy, risk management and tourism industry.
A long-term WRF simulation data set in the UAE area from a project funded by AGEDI (Abu Dhabi Global Environmental Data Initiative) as part of EAD (Environment Agency Abu Dhabi) has been analyzed by the team. This AGEDI data set is compared to available observations and the new regional climate simulation data. Model uncertainties can be estimated based on multiple data sets.
This work addresses the objectives 5 and 6 and serves as the basis for objective 3.
Idealized and real case cloud seeding simulations
The proposed idealized seeding runs will be simulated by both the bulk and bin schemes. The cloud seeding and sensitivity real case simulations will be conducted over a domain covering the interested event with a grid spacing of 500-m with the purpose to explicitly resolve most of the convective systems including both deep and shallow convections and the fine-scale interaction between the topography and the flow. These simulations will be driven by the regional climate simulation results. We will select appropriate cases from the UAEREP funded field campaigns to conduct hygroscopic and/or glaciogenic seeding simulations using mostly the bulk scheme. For both idealized and real case seeding simulations, different environmental conditions such as CCN and IN concentrations, model physics and seeding parameterizations will be tested. The piggybacking approach will provide quantitative answers to how cloud seeding or other mechanisms impact rainfall through microphysical and dynamical processes individually and jointly. Different seeding and sensitivity simulations of the same cases working as ensemble members will provide uncertainties or the range of their effects on rainfall. These simulations will address objective 2.